The Elusive Dream: Room-Temperature Superconductors and the Quest for Energy Efficiency
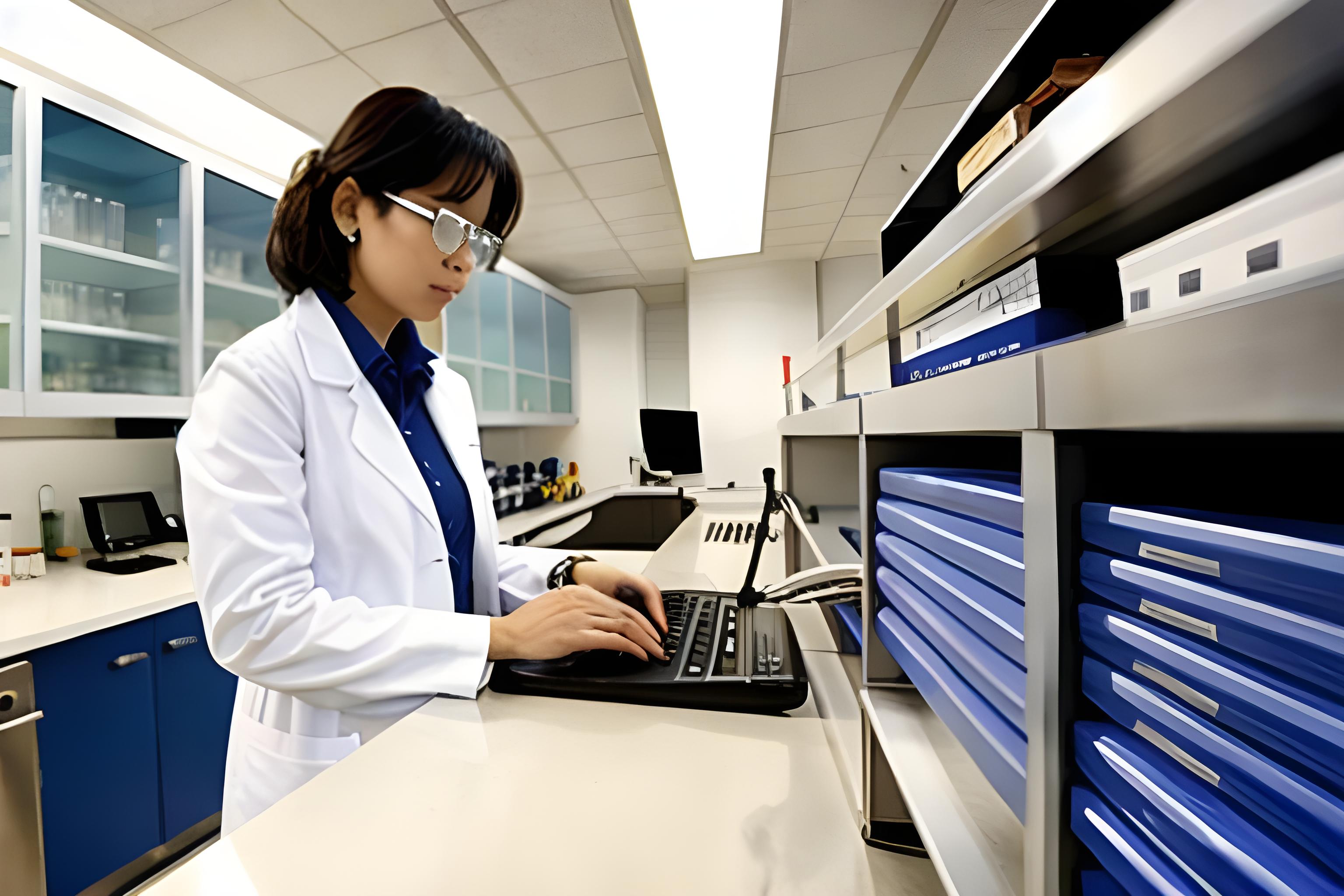
1 Introduction
When Dutch physicist Heike Kamerlingh Onnes first discovered superconductivity in mercury at ultra-low temperatures back in 1911, little did he know that he was laying the groundwork for an endless journey of scientific investigation. Superconductors, with their ability to carry an electric current without any resistance, hold an irresistible allure that has challenged the scientific community for over a century. This potential for a lossless transmission of energy carries transformative implications for the realms of electricity generation, distribution, and storage. However, the most tantalizing prospect of all is the development of superconductors that can function at room temperature. This has remained an elusive dream for physicists and material scientists across the globe, a monumental scientific puzzle that has yet to be solved as of 2023.
2 Understanding Superconductivity
Superconductivity is a unique quantum mechanical phase that occurs in certain materials when cooled to extremely low temperatures. This transition to a superconducting state is characterized by a sudden drop in electrical resistance to precisely zero, allowing for the perpetuation of electrical current indefinitely in the absence of external power. This phenomenon, when first discovered, was a radical contradiction to classical physics, which held that all materials inevitably resist the flow of electrical current to some degree.
At the core of superconductivity is the formation of Cooper pairs, named after physicist Leon Cooper. A Cooper pair consists of two electrons with opposite momenta and spins, which form in the low-temperature environment. These pairs of electrons can move through the superconducting material without scattering off impurities or defects – the typical causes of resistance in normal conductors. This understanding of superconductivity earned Leon Cooper and his colleagues, John Bardeen and John Robert Schrieffer, the Nobel Prize in Physics in 1972, for what is now known as the BCS Theory (Bardeen–Cooper–Schrieffer Theory).
3 The High-Temperature Conundrum
Early superconductors, like mercury used in Onnes’s experiments, had to be cooled to within a few degrees of absolute zero (-273.15 degrees Celsius) to achieve this state, using costly and inefficient liquid helium. It wasn’t until the 1980s when IBM researchers Georg Bednorz and K. Alex Müller discovered the first high-temperature superconductors (HTS) - a class of ceramic materials known as cuprates. This breakthrough, which earned them a Nobel Prize in 1987, paved the way for the discovery of other HTS materials that could superconduct at temperatures as “high” as -135 degrees Celsius, achievable with the cheaper and more plentiful liquid nitrogen.
Yet, despite these advancements, the real prize - room-temperature superconductivity - has remained just out of reach. The reasons for this are twofold: the complex nature of the materials involved, and the limitations of our current understanding of the underlying physics.
4 Complexity of Materials
High-temperature superconductors, including cuprates and more recently discovered iron-based superconductors and hydrogen-rich materials, are often very complex, structurally disordered materials. They contain multiple elements and exhibit numerous phases, making them difficult to manufacture and characterize. Moreover, the exact mechanisms behind their superconducting behavior differ from the traditional BCS theory, involving strong electron correlations and exotic quantum states. This complexity makes it challenging to predict new superconducting materials and to improve their properties systematically.
5 Gaps in Understanding
The phenomenon of superconductivity, particularly in high-temperature superconductors, is still not fully understood. While the BCS theory explains low-temperature superconductivity well, it fails to adequately explain why certain materials can become superconducting at higher temperatures. New theories have been proposed, often involving intricate quantum mechanics and complex interactions between electrons, but a comprehensive, universally accepted explanation remains elusive. This lack of understanding is a major barrier to the intentional design of room-temperature superconductors.
6 The Road Ahead
Scientists and researchers are using advanced techniques, from high-pressure physics to machine learning algorithms, in the quest for room-temperature superconductivity. Progress is being made incrementally. In 2020, for example, researchers at the University of Rochester reported superconductivity at room temperature in a material composed of carbon, sulfur, and hydrogen - albeit at extreme pressures of around 270 gigapascals, about a million times higher than typical atmospheric pressure.
The pursuit of room-temperature superconductivity is a fascinating scientific journey, one that intertwines the boundaries of physics, materials science, and engineering. With every new discovery and every incremental increase in operating temperature, we move one step closer to realizing the profound potential of this revolutionary technology. It’s a tantalizing prospect, promising vast improvements in energy efficiency, transportation, computing, and more. But until the dream is fully realized, the quest for room-temperature superconductivity remains one of the most thrilling and challenging endeavors in modern science.